Cracking OS X Lion Passwords
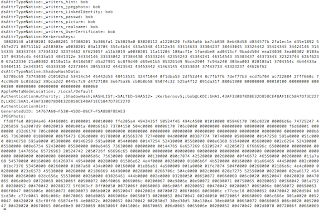
UPDATE [2011-10-15]: The issues described in this post have now been resolved by Apple. Users running OS X Lion 10.7.2 or security update 2011-006 are no longer affected by the vulnerabilities detailed below (CVE-2011-3435 and CVE-2011-3436). For further details on this security update please see Apple's advisory . In 2009 I posted an article on Cracking Mac OS X passwords . Whilst this post has been quite popular, it was written for OS X 10.6 and prior. Since the release of Mac OS X Lion (10.7) in July, I have received numerous requests for an update. Typically, I would have just updated the existing article without the need for a new post. However, during my research I discovered something interesting about OS X Lion that I'd like to share. In previous versions of OS X (10.6, 10.5, 10.4) the process to extract user password hashes has been the same: obtain the user's GeneratedUID and then use that ID to extract hashes from a specific user's shadow file (See my pr